TOP: A huge star-forming region is rotating globally (white arrow). This large region can make multiple stellar systems. MIDDLE: Three protostars form deep in the collapsing cloud. The collapse causes eddies, allowing newly-forming stars to rotate in different directions and at different speeds (see arrows). BOTTOM: One protostellar cloud collapses further into a disk-like structure that rotates counter-clockwise (white arrows) about the newly-formed protostar. The protostar siphons material from a passing protostellar cloud rotating in the opposite direction, causing the outer part of the disk to rotate clockwise (yellow arrows). Eventually, planets will form, with the outer planets orbiting the star in the opposite direction from the inner planets.
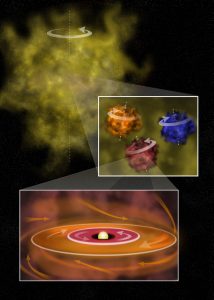
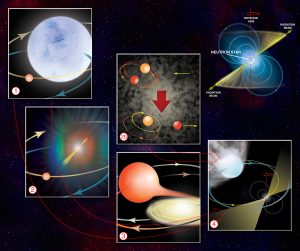
How to Make a Millisecond Pulsar
The diagram outlines each step needed to create a pulsar with a superfast spin. The Standard Scenario (1-4):
1. A massive supergiant star and a “normal” Sun-like star orbit each other in a binary star system.
2. The massive star exhausts its nuclear fuel and explodes in a supernova, leaving a neutron star as its remnant. For several tens of millions of years, that neutron star is likely active as a radio pulsar. Eventually it slows down, turns off as a pulsar and becomes simply a cooling neutron star.
3. After billions of years, and if the binary survived the supernova, the lower-mass star evolves and expands into a red giant. Material from the star spills into a disk around the neutron star and eventually onto the neutron star surface in a process known as “accretion.” Accretion transfers angular momentum to the neutron star and makes it spin more rapidly. During this process, the system is visible as an X-ray binary.
4. Once accretion ends, the neutron star is spinning very rapidly and emerges as a millisecond radio pulsar. This process is known as “recycling.” Strong “winds” from the pulsar slowly erode away the companion star, which can eclipse the pulsar’s radio emission.
But in a globular cluster (2b)…
There are so many stars packed so densely together that interactions between ancient “dead” neutron stars and normal stellar binaries can occur (top). When that happens, in a process called “exchange,” the lowest mass star is usually ejected from the binary and the neutron star replaces it (bottom). Once the remaining normal star evolves into a red giant, the rest of the standard “recycling” scenario (3-4) takes place. These interactions and exchanges explain why globular clusters have so many more millisecond pulsars per unit mass than the Galactic disk.
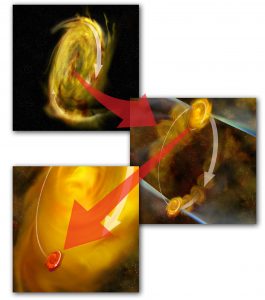
Disks Inside Disks
An artist’s interpretation of a radio object known as L1551 IRS5, which is two young star systems, still surrounded by a disk of gas and dust, in orbit around each other.
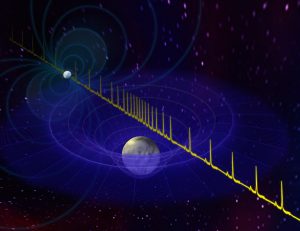
How to Weigh a Pulsar
Pulses from a neutron star (rear) are slowed as they pass near the foreground white dwarf star. The effect, known as the Shapiro Delay, is the extra time delay light experiences by traveling past a massive object due to general relativistic time dilation. Spinning 317 times per second, this pulsar (PSR J1614-2230) and its companion white dwarf star complete an orbit in just under nine days. The pair, some 3,000 light-years distant, are in an orbit seen almost exactly edge-on from Earth, which gives astronomers the information they need to make a mass measurement.
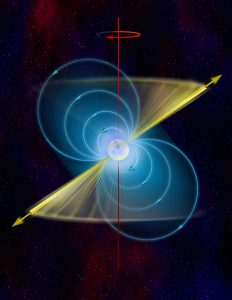
Pulsar
In this artist’s interpretation, the basics of a pulsar are color-coded. In white is the neutron star. Its powerful magnetic field is shown in blue. The north and south poles of that magnetic field, and the directions from which the pulsar’s beams shoot, are in yellow. And in red is the axis of rotation of the star. That axis is offset from the beam, which is why when the star spins, the beam sweeps past us.
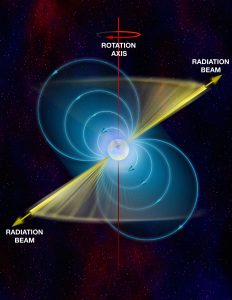
Parts of a Pulsar
In this artist’s interpretation, the basics of a pulsar are color-coded. In white is the neutron star. Its powerful magnetic field is shown in blue. The north and south poles of that magnetic field, and the directions from which the pulsar’s beams shoot, are in yellow. And in red is the axis of rotation of the star. That axis is offset from the beam, which is why when the star spins, the beam sweeps past us.