EHT Newsroom: Quest for the Shadow of a Black Hole
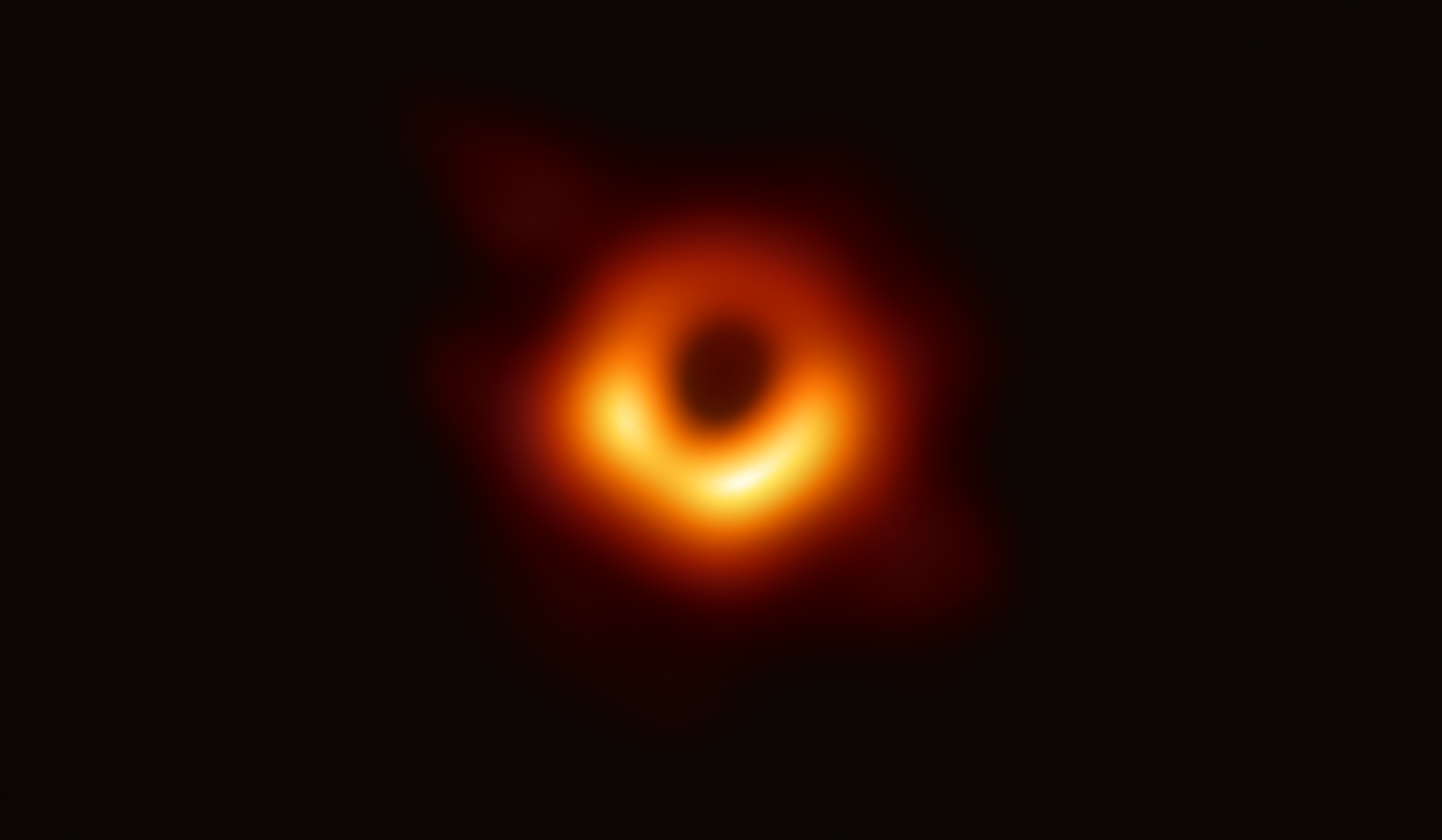
Astronomers Capture First Image of a Black Hole
Image Credit: EHT Collaboration
The Event Horizon Telescope (EHT) — a planet-scale array of eight ground-based radio telescopes forged through international collaboration — was designed to capture images of a black hole. Today, in coordinated press conferences across the globe, EHT researchers reveal that they have succeeded, unveiling the first direct visual evidence of a supermassive black hole and its shadow.
This breakthrough was announced today in a series of six papers published in a special issue of The Astrophysical Journal Letters. The image reveals the black hole at the center of Messier 87, a massive galaxy in the nearby Virgo galaxy cluster. This black hole resides 55 million light-years from Earth and has a mass 6.5-billion times that of the Sun.
The EHT links telescopes around the globe to form an Earth-sized virtual telescope with unprecedented sensitivity and resolution. The EHT is the result of years of international collaboration, and offers scientists a new way to study the most extreme objects in the Universe predicted by Einstein’s general theory of relativity during the centennial year of the historic experiment that first confirmed the theory.
“We have taken the first picture of a black hole,” said EHT project director Sheperd S. Doeleman of the Center for Astrophysics | Harvard & Smithsonian. “This is an extraordinary scientific feat accomplished by a team of more than 200 researchers.”
Black holes are extraordinary cosmic objects with enormous masses but extremely compact sizes. The presence of these objects affects their environment in extreme ways, warping spacetime and super-heating any surrounding material.
“If immersed in a bright region, like a disk of glowing gas, we expect a black hole to create a dark region similar to a shadow — something predicted by Einstein’s general relativity that we’ve never seen before,” explained chair of the EHT Science Council Heino Falcke of Radboud University, the Netherlands. “This shadow, caused by the gravitational bending and capture of light by the event horizon, reveals a lot about the nature of these fascinating objects and allowed us to measure the enormous mass of M87’s black hole.”
Multiple calibration and imaging methods have revealed a ring-like structure with a dark central region — the black hole’s shadow — that persisted over multiple independent EHT observations.
“Once we were sure we had imaged the shadow, we could compare our observations to extensive computer models that include the physics of warped space, superheated matter and strong magnetic fields. Many of the features of the observed image match our theoretical understanding surprisingly well,” remarks Paul T.P. Ho, EHT Board member and Director of the East Asian Observatory [5]. “This makes us confident about the interpretation of our observations, including our estimation of the black hole’s mass.”
Creating the EHT was a formidable challenge that required upgrading and connecting a worldwide network of eight pre-existing telescopes deployed at a variety of challenging high-altitude sites. These locations included volcanoes in Hawai`i and Mexico, mountains in Arizona and the Spanish Sierra Nevada, the Chilean Atacama Desert, and Antarctica.
The EHT observations use a technique called very-long-baseline interferometry (VLBI) which synchronises telescope facilities around the world and exploits the rotation of our planet to form one huge, Earth-size telescope observing at a wavelength of 1.3mm. VLBI allows the EHT to achieve an angular resolution of 20 microarcseconds — enough to read a newspaper in New York from a sidewalk café in Paris.
The telescopes contributing to this result were ALMA, APEX, the IRAM 30-meter telescope, the James Clerk Maxwell Telescope, the Large Millimeter Telescope Alfonso Serrano, the Submillimeter Array, the Submillimeter Telescope, and the South Pole Telescope [7]. Petabytes of raw data from the telescopes were combined by highly specialised supercomputers hosted by the Max Planck Institute for Radio Astronomy and MIT Haystack Observatory.
The construction of the EHT and the observations announced today represent the culmination of decades of observational, technical, and theoretical work. This example of global teamwork required close collaboration by researchers from around the world. Thirteen partner institutions worked together to create the EHT, using both pre-existing infrastructure and support from a variety of agencies. Key funding was provided by the US National Science Foundation (NSF), the EU’s European Research Council (ERC), and funding agencies in East Asia.
“We have achieved something presumed to be impossible just a generation ago,” concluded Doeleman. “Breakthroughs in technology, connections between the world’s best radio observatories, and innovative algorithms all came together to open an entirely new window on black holes and the event horizon.”
“When NSF established the NRAO in 1956, it laid the groundwork for decades of discovery,” said astrophysicist Joe Pesce, who oversees NRAO’s NSF funding. “Today, the fruits of that investment are particularly evident in a truly remarkable achievement of observational astronomy.”
“Through its leadership in ALMA and long-term support for the EHT, NRAO has once again helped to advance our understanding of the cosmos and the fundamental laws physics. This observation clearly illustrates the value of radio astronomy to scientific advancement. The next generation of radio telescopes, including the Next Generation VLA, will yield many more groundbreaking results,” said NRAO Director Tony Beasley.
# # #
Notes
[1] The shadow of a black hole is the closest we can come to an image of the black hole itself, a completely dark object from which light cannot escape. The black hole’s boundary — the event horizon from which the EHT takes its name — is around 2.5 times smaller than the shadow it casts and measures just under 40 billion km across.
[2] Supermassive black holes are relatively tiny astronomical objects — which has made them impossible to directly observe until now. As a black hole’s size is proportional to its mass, the more massive a black hole, the larger the shadow. Thanks to its enormous mass and relative proximity, M87’s black hole was predicted to be one of the largest viewable from Earth — making it a perfect target for the EHT.
[3] Although the telescopes are not physically connected, they are able to synchronize their recorded data with atomic clocks — hydrogen masers — which precisely time their observations. These observations were collected at a wavelength of 1.3 mm during a 2017 global campaign. Each telescope of the EHT produced enormous amounts of data – roughly 350 terabytes per day – which was stored on high-performance helium-filled hard drives. These data were flown to highly specialised supercomputers — known as correlators — at the Max Planck Institute for Radio Astronomy and MIT Haystack Observatory to be combined. They were then painstakingly converted into an image using novel computational tools developed by the collaboration.
[4] 100 years ago, two expeditions set out for the island of Príncipe off the coast of Africa and Sobral in Brazil to observe the 1919 solar eclipse, with the goal of testing general relativity by seeing if starlight would be bent around the limb of the sun, as predicted by Einstein. In an echo of those observations, the EHT has sent team members to some of the world’s highest and isolated radio facilities to once again test our understanding of gravity.
[5] The East Asian Observatory (EAO) partner on the EHT project represents the participation of many regions in Asia, including China, Japan, Korea, Taiwan, Vietnam, Thailand, Malaysia, India and Indonesia.
[6] Future EHT observations will see substantially increased sensitivity with the participation of the IRAM NOEMA Observatory, the Greenland Telescope and the Kitt Peak Telescope.
[7] ALMA is a partnership of the European Southern Observatory (ESO; Europe, representing its member states), the U.S. National Science Foundation (NSF), and the National Institutes of Natural Sciences (NINS) of Japan, together with the National Research Council (Canada), the Ministry of Science and Technology (MOST; Taiwan), Academia Sinica Institute of Astronomy and Astrophysics (ASIAA; Taiwan), and Korea Astronomy and Space Science Institute (KASI; Republic of Korea), in cooperation with the Republic of Chile. APEX is operated by ESO, the IRAM 30-meter telescope is operated by IRAM (the IRAM Partner Organizations are MPG (Germany), CNRS (France) and IGN (Spain)), the James Clerk Maxwell Telescope is operated by the EAO, the Large Millimeter Telescope Alfonso Serrano is operated by INAOE and UMass, the Submillimeter Array is operated by SAO and ASIAA and the Submillimeter Telescope is operated by the Arizona Radio Observatory (ARO). The South Pole Telescope is operated by the University of Chicago with specialized EHT instrumentation provided by the University of Arizona.
More Information
This research was presented in a series of six papers published today in a special issue of The Astrophysical Journal Letters.
The EHT collaboration involves more than 200 researchers from Africa, Asia, Europe, North and South America. The international collaboration is working to capture the most detailed black hole images ever by creating a virtual Earth-sized telescope. Supported by considerable international investment, the EHT links existing telescopes using novel systems — creating a fundamentally new instrument with the highest angular resolving power that has yet been achieved.
The individual telescopes involved are; ALMA, APEX, the IRAM 30-meter Telescope, the IRAM NOEMA Observatory, the James Clerk Maxwell Telescope (JCMT), the Large Millimeter Telescope Alfonso Serrano (LMT), the Submillimeter Array (SMA), the Submillimeter Telescope (SMT), the South Pole Telescope (SPT), the Kitt Peak Telescope, and the Greenland Telescope (GLT).
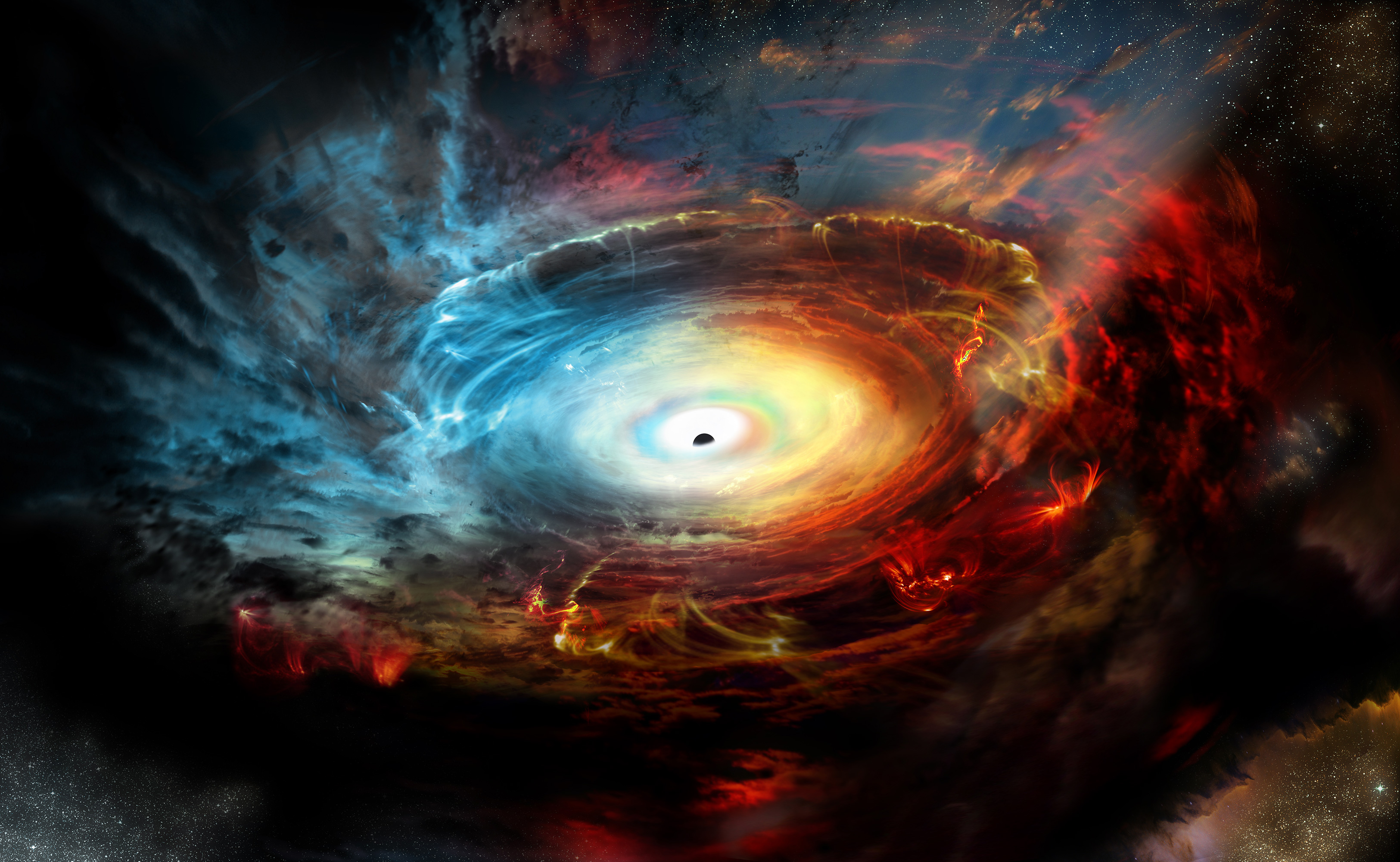
The Event Horizon of a Black Hole: A “Grand Challenge” of Astronomy
At the edge of observable space where gravity becomes so strong that it literally twists the fabric of the universe, science predicts some seemingly impossible phenomena – the warping of light, the distortion of time and space, and a point of no return where matter and energy are forever lost.
This is the so-called “event horizon” of a black hole. When surrounded by a hot disk of gas and dust, the event horizon should become visible, appearing as a dark shadow against a glowing background. It was the EHT’s goal to image this feature and study its remarkable behavior.
Astronomers have all but proven the existence of supermassive black holes, albeit indirectly. Images of powerful radio jets blasting out of galactic cores and ongoing studies of stars careening around the center of the Milky Way clearly herald the presence of a supermassive black hole. But Einstein’s general theory of relatively tells us there is more concrete evidence, if we could only see the black hole’s direct gravitational influence on the nearby fabric of spacetime.
Though massive beyond comprehension, a galaxy’s central black hole is so compact that its gravity dominates only a relatively small region near the center of the galaxy. Until now, a black hole’s relatively small size has thwarted up-close observations of a black hole’s event horizon, even with the most advanced telescopes. Adding to the challenge, most supermassive black holes are embedded deep within thick regions of dust and gas, which completely obscure them from view by optical telescopes. The Event Horizon Telescope, sometimes called “Astronomy’s Killer App,” has overcome both these challenges.
Image Credit: NRAO/AUI/NSF; D. Berry
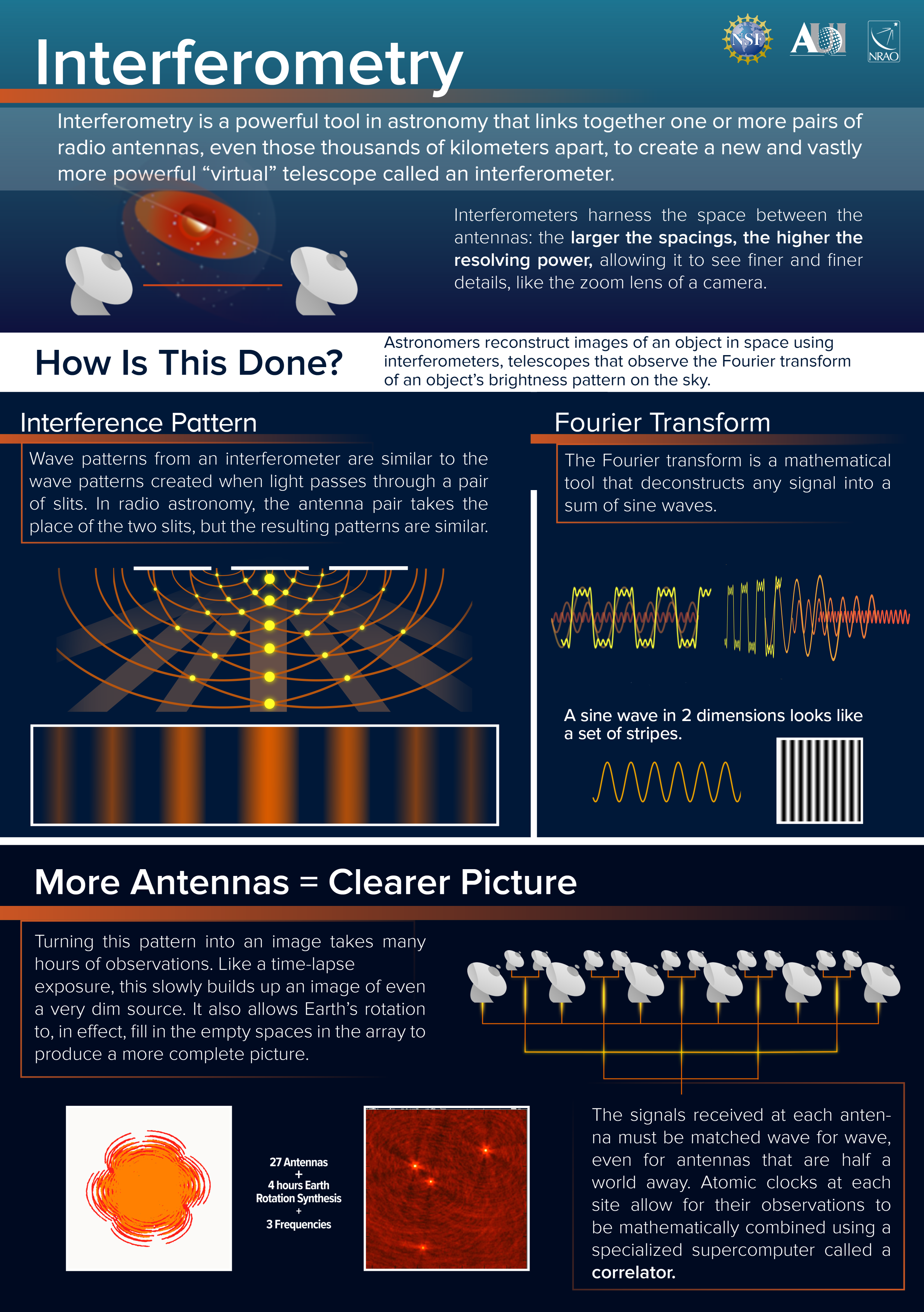
Interferometers: Telescopes Made Mostly of Nothing
Radio telescopes like the EHT can study the universe in ways that are beyond the reach of optical telescopes. Radio telescopes can see the light naturally emitted by molecules and cold dust. Radio light, which has longer wavelengths than visible light, can pass through the interstellar dust and gas, revealing details at the centers of galaxies.
Longer wavelengths of light, however, pose additional challenges to observers on Earth. To see objects at radio wavelengths, astronomers require vastly larger telescopes than are needed for similar visible-light observations. For example, to see with the same clarity as the 2.4 meter Hubble Space Telescope, radio observations at a relatively short 1-centimeter wavelength would need a dish 20 kilometers in diameter. It would be all-but-impossible to build such a telescope, yet astronomers have found a way around this limitation, achieving a resolution far beyond that of Hubble: radio interferometry.
Interferometry is a basic principle in physics and a powerful tool in astronomy. With it, scientist are able to link together one or more pairs of radio antennas, even those thousands of kilometers apart, to create a new and vastly more powerful “virtual” telescope.
Imagine drawing a line between a pair of antennas; astronomers call this a “baseline.” The longer the baseline, the larger the aperture of the virtual telescope. The larger the aperture, the greater its resolution.
The high resolution is possible thanks to the ability of light waves to overlap and merge with each other, creating a distinctive wave-like interference pattern.
Interference patterns are easy to see. All you need is a light source, a dark room, and a barrier with two distinct openings, or slits. After the light passes through the slits it overlaps and combines to create alternating light and dark bands.
In the case of a radio interferometer, the two antennas act like slits, capturing waves of radio light from the same cosmic source. If the antennas are too far apart to connect with a wire or cable (one in Hawaii and one in the Caribbean, to use a real-world example), astronomers record and precisely time-stamp the signals from each antenna. These recordings are then brought together and combined using powerful computers. Astronomers can then use a mathematical tool called a Fourier transform to reconstruct an image of a cosmic object as it would be seen in radio light.
Image credit: NRAO/AUI/NSF; S. Dagnello

One Pair, Two Pairs, Any Pair to Many Pairs
A single pair of antennas, however, is not an effective imaging tool. More linked antenna pairs, known as an array, are necessary to form a clearer picture.
In an array, every antenna forms a baseline with each other antenna. For example, the 27 dishes of the National Science Foundation’s Very Large Array create 351 baselines. (Adding just one more antenna would increase the number of baselines to 378.)
Turning this many-baseline image into a truly accurate picture, however, requires one more ingredient: time. Like a time-lapse exposure, radio telescopes require time to build-up an image of very dim sources. Time also allows the Earth to rotate, effectively “filling in the gaps ” to produce a more complete picture, a process astronomers call “aperture synthesis.”
Arrays like the VLA and ALMA use fiber optic cable to transmit the vast amounts of data from their antennas. That technology allows for baselines tens-of-kilometers long. At these distances and depending on the frequency, the VLA can achieve a resolution of 0.04 arcseconds, which is about as sharp as the Hubble Space Telescope.
Interferometry need not stop there, however. Very long baselines, hundreds to even thousands of kilometers long, are possible even when fiber optic connections are not practical. In these cases, astronomers record the signals and timecode the data using precise atomic clocks. The data is then shipped to a central location where the signals can be matched, wave for wave, by special-purpose computers called correlators. So long as the signals are received in good order at all the sites and are properly timecoded, extreme resolutions like those achieved by the EHT are possible. This is, in principle, very simple, but it is daunting in real life.
Each antenna must observe the same object at precisely the same time and at the exact same frequency. In addition, each site must have suitable weather conditions for clear observations. Even then, the target of observations may not be visible or only visible to a portion of the EHT sites.
Image Credit: NRAO/AUI/NSF; J. Hellerman
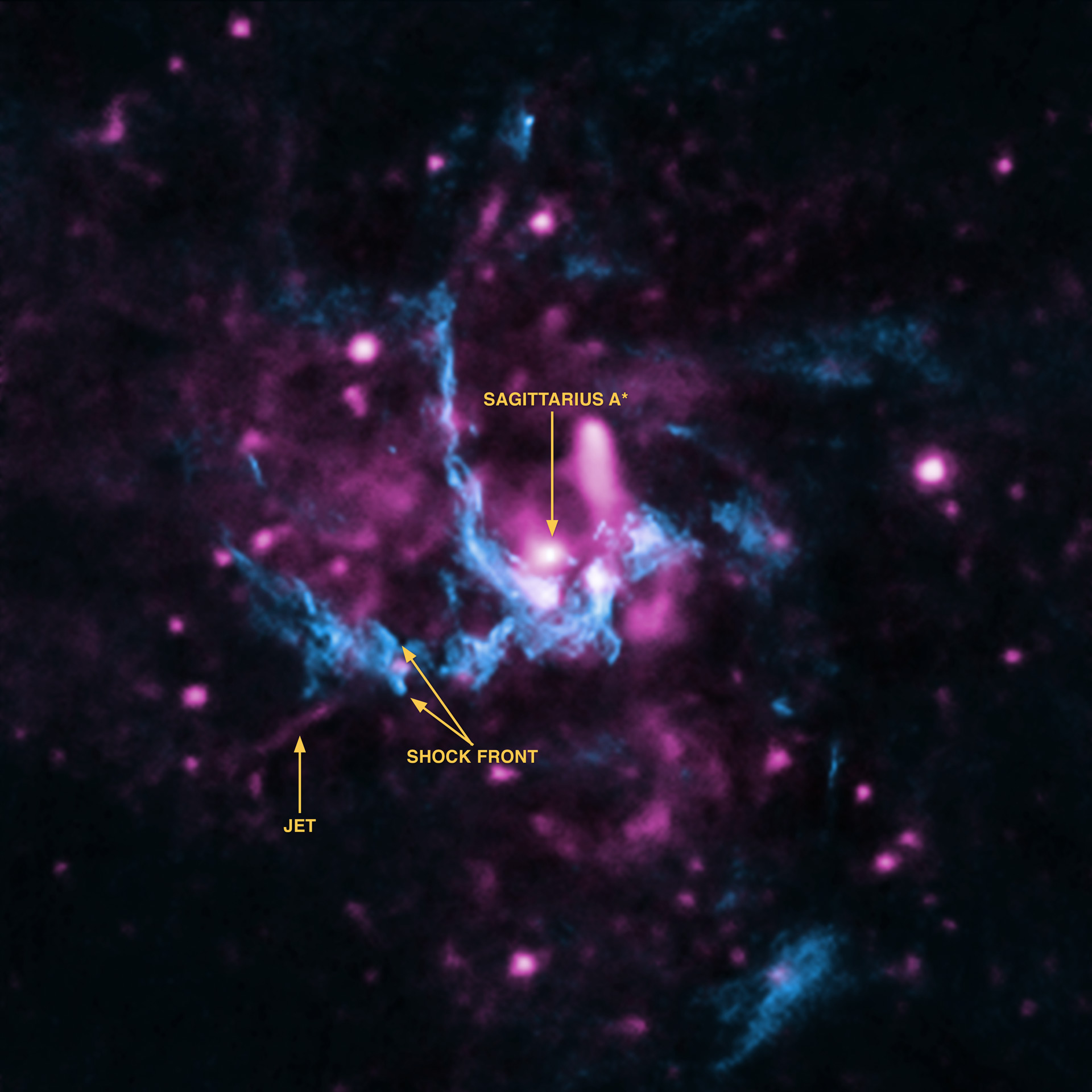
Black Hole at the Center of the Milky Way Launches Radio Astronomy
Radio astronomy began in the early 1930s when a young engineer named Karl Jansky was asked to investigate sources of interference that might hinder transatlantic shortwave radio communications. Using a simple directional antenna, Jansky discovered an unexpected source of static coming from the constellation Sagittarius, which is in the general direction of the center of the Milky Way. This discovery was widely publicized, appearing in the New York Times in 1933. Though a curiosity, few people at the time gave it much thought and none recognized that this strange signal was coming from a supermassive black hole.
With advances in radio technology developed during the Second World War, astronomers began to acquire the sensitivity and resolution necessary to pick up where Jansky left off and explore the cosmos at radio wavelengths. The first being amateur astronomer and ham radio operator Grote Reber.
In 1959, the National Radio Astronomy Observatory at Green Bank, West Virginia, was already conducting pioneering science with an 85-foot diameter radio telescope called the Tatel Telescope. In 1964, the scientific staff there built a twin, 85-foot telescope — cleverly named 85-2. These two antennas could be linked – combining the signal of one with the signal of the other to create the first interferometer in the United States. Unlike modern interferometers that used digitized signals, the Green Bank experiment used copper-lined tube to directly transmit the analog signals from the antennas. By hauling 85-2 closer or further, the astronomers could change the pair’s baseline, altering its resolution.
A third 85-foot dish was added in 1966, along with a portable 45-foot antenna that was located at distances up to 35 kilometers away and was connected to the other three elements by a radio link. This Green Bank Interferometer became the testbed for what would eventually become the iconic Very Large Array in New Mexico.
The Green Bank Interferometer (GBI) was not solely a VLA testbed, however. It was a fully functioning, state-of-the-art interferometer on its own. Most notably, in mid-February of 1974, the GBI discovered an intense and point-like source of radio waves coming from the heart of our Milky Way Galaxy, the radio source Sagittarius A*. It was an incredibly compact object and soon determined to be a supermassive black hole.
The GBI continued scientific observations until 1978. Among its many results was the first radio measurement that confirmed, to high accuracy, Einstein’s prediction of the bending of light (i.e. any electromagnetic radiation) near a massive body, commonly called Einstein rings or gravitational lensing.
With the VLA, the Very Long Baseline Array, and ALMA, astronomers have harnessed the potential of interferometry, yielding new science both independently and in collaboration with other telescopes both on Earth and in space.

VLBI: Timing Is Key
Radio waves from distant celestial objects arrive at different points on Earth at different times. For VLBI to work, these waves must be matched wave-for-wave at each and every station. To accomplish this, the EHT uses ultra-precise atomic clocks, which time-stamp the data.
Later, the data are shipped via hard drives to a centralized location where astronomers combine the signals using a supercomputer known as a correlatorCorrelatorA specialized supercomputer that multiplies the data from two antennas and averages the result over time. Essentially the correlator only selects the data that is spotted and analyzed by both antennas, which means the data that isn’t spotted by both is dropped. to effectively create an Earth-size telescope and produce remarkably high-resolution images.
This graphic illustrates how the radio light from distant cosmic objects arrive at the various EHT stations at different times.
Image Credit: NRAO/AUI/NSF, S. Dagnello

VLA Shows Power of Supermassive Black Hole in M87
Long before the EHT, NRAO telescopes studied the black hole at the heart of M87.
In 1999, researchers using the National Science Foundation’s Very Large Array (VLA) radio telescope imaged a “spectacular and complex structure” of galaxy M87, which harbors at its core a supermassive black hole spewing out jets of subatomic particles at nearly the speed of light and also is the central galaxy of the Virgo Cluster of galaxies.
The VLA image is the first to show detail of a larger structure that originally was detected by radio astronomers more than a half-century ago. The observations show two large, bubble-like lobes, more than 200,000 light-years across, that emit radio waves. These lobes, which are intricately detailed, apparently are powered by gravitational energy released from the black hole at the galaxy’s center.
M87, discovered by the French astronomer Charles Messier in 1781, is the strongest radio-emitting object in the constellation Virgo. Its jet was described by Lick Observatory astronomer Heber Curtis in 1918 as “a curious straight ray … apparently connected with the nucleus by a thin line of matter.” In 1954, Walter Baade reported that the jet’s light is strongly polarized. M87’s X-ray emission was discovered in 1966. M87 is the largest of the thousands of galaxies in the Virgo Cluster. The Local Group of galaxies, of which our own Milky Way is one, is part of the Virgo Cluster’s outskirts.
The galaxy’s radio emissions first were observed by Australian astronomers in 1947, but the radio telescopes of that time were unable to discern much detail. They could, however, show that there is a structure more than 100,000 light-years across.
Subsequent radio images, particularly those made using the sharp radio “vision” of the VLA, were primarily aimed at studying the inner 10,000 light-years or so, and showed great detail in the galaxy’s jet. Astronomers even have followed the motions of concentrations of material within the jet over time. These observations, however, did not show much about the larger structure that was seen by earlier radio astronomers, leaving its details largely a mystery.
The mystery was solved by using the VLA to observe at longer radio wavelengths, thus revealing larger-scale structures.
The VLA Astronomers have captured the first direct visual evidence of a black holeBlack HoleAn object – typically a collapsed star – whose gravity is so strong that its escape velocity exceeds the speed of light.: an image of the supermassive black holeSupermassive Black HoleA black hole that has a million or as much as a billion solar masses. These large black holes lurk at the centers of most galaxies. at the center of Messier 87 (M87), a giant elliptical galaxyElliptical GalaxyA galaxy that appears round or elliptical in shape, typically containing little gas and dust, no spiral arms or disk, mostly older stars, which range in shape from spherical to "football" shaped, and few hot, bright stars. 53 million light-yearsLight-yearsThe distance that light travels in one year in a vacuum. One light year is equivalent to about six trillion miles. from Earth. This unprecedented observation was made possible by the Event Horizon Telescope (EHT), an array of eight individual radio telescopes spread over four continents and linked together to form a new, exceptionally powerful telescope.
Credit: NRAO/AUI/NSF

NSF's VLBA: Essential to Studies of Supermassive Black Holes
Additional important discoveries include:
VLBA Observations Reveal Tremendous Mass Concentration
at the Heart of Strange Galaxy: While astronomers had long suspected that supermassive black holes, millions or billions of times more massive than our Sun, lurked at the cores of powerful radio galaxies, the National Science Foundation’s Very Long Baseline Array (VLBA)Very Long Baseline Array (VLBA)An array of 10, 25 meter radio telescopes that stretches 8000km (5,000 miles) across North America. has provided some of the most compelling quantitative evidence that this is indeed the case. An international team of researchers announced this conclusion in a paper published in the scientific journal Nature in 1995.
VLBA Reveals Cosmic Jet Formation: Astronomers have gained their first glimpse of the mysterious region near a black hole at the heart of a distant galaxy, where a powerful stream of subatomic particles spewing outward at nearly the speed of light is formed into a beam, or jet, that then goes nearly straight for thousands of light-years. The results, released in 1999, show that M87’s jet is formed within a few tenths of a light-year of the galaxy’s core, presumed to be a black hole three billion times more massive than the sun. In the formation region, the jet is seen opening widely, at an angle of about 60 degrees, nearest the black hole, but is squeezed down to only 6 degrees a few light-years away.
VLBA Locates Superenergetic Bursts: A giant galaxy’s bursts of very high energy gamma rays are coming from a region very close to the supermassive black hole at its core. The discovery provides important new information about the mysterious workings of the powerful “engines” in the centers of innumerable galaxies throughout the Universe.
Approaching the Black Hole: Astronomers Pinpointed the Location of the Central Black Hole in M87
Using the NSF’s VLBA, researchers peered through the upper stream of the jet and the heart of M87 pulsing as their central super massive black hole. M87 is the largest galaxy in the Virgo Cluster of galaxies and one of the closest examples of the powerful radio jets. With the VLBA’s super eye, the researchers pinpoint the exact origin of the jet where the black hole resides in M87. The determination of the location of the black hole provides important new information about the mysterious about workings of the powerful “engines” in the centers of innumerable galaxies throughout the Universe.
Image Credit: NRAO/AUI/NSF, See corresponding news release
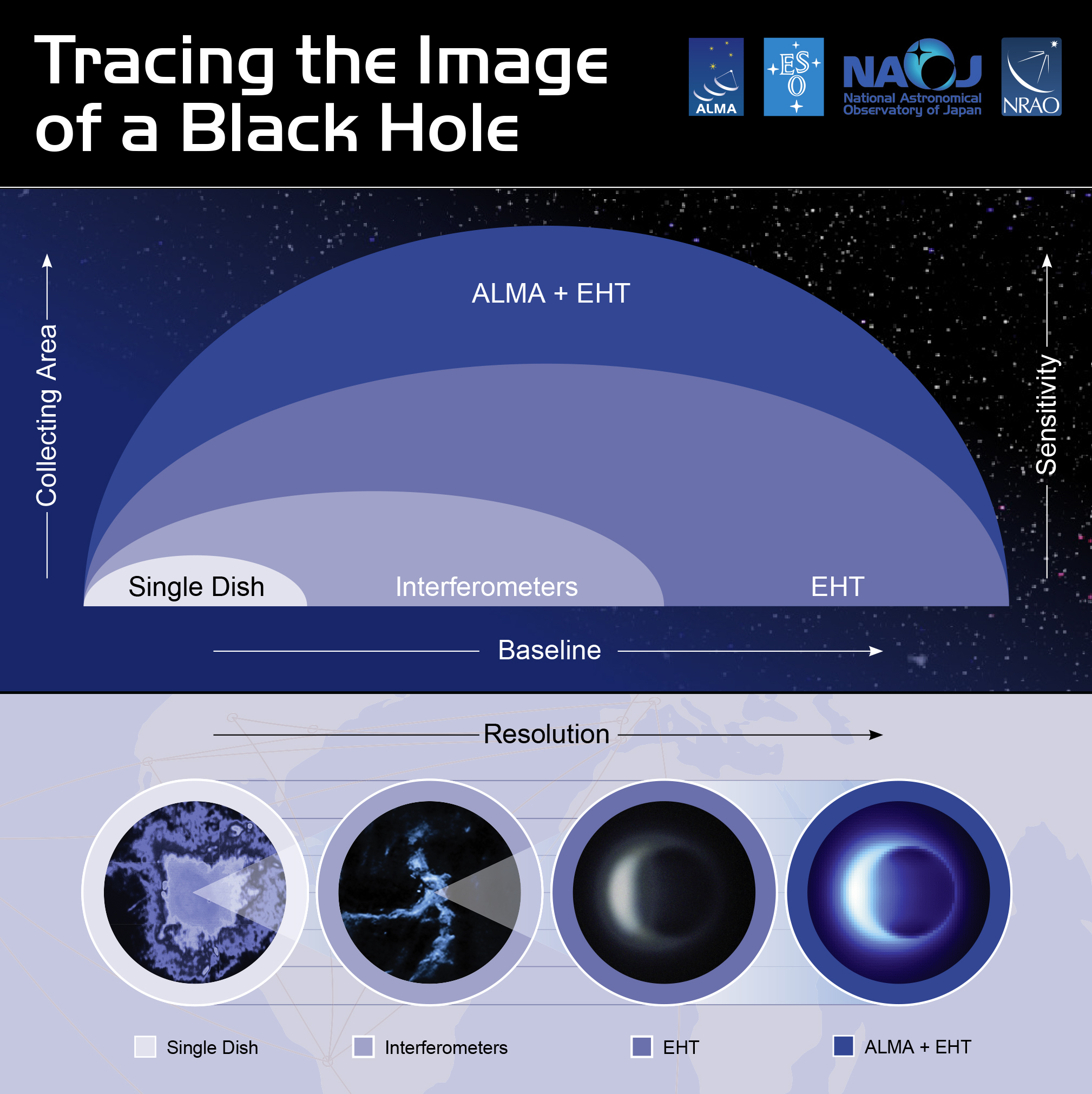
Adding ALMA to the EHT
ALMA is an interferometer with 66 antennas and baselines up to 16 kilometers long. To join the EHT, however, ALMA had to operate differently. It had to become a phased array, which would be like smooshing all the antennas together into one single dish. To make this change, specialized electronics and computer equipment were built at the National Radio Astronomy Observatory’s Central Development Lab in Charlottesville, Virginia, and then installed in ALMA’s correlator, the supercomputer that combines the signals from the antennas.
The signal from the phased array were then time-stamped and encoded by a dedicated atomic clock – the new hydrogen maser procured and tested by MIT’s Haystack Observatory. There data were then shipped to a central processing center and combined with identically timed signals from other telescopes.
Image Credit: NRAO/AUI/NSF; N. Janney/J. Hellerman