Take a virtual trek of the Milky Way Galaxy in exclusive video tours with our experts — Visit the Milky Way Explorer!
On a clear, dark night, you can see a glowing stream that seems to split the sky. We have called it the Milky Way Galaxy for thousands of years, and its exact nature was a mystery until less than a hundred years ago.
Although early telescopes resolved the Milky Way Galaxy into individual stars, we needed to measure the distances to and motions of these stars before a three-dimensional picture emerged: The band of stars has a depth, it encircles us, and the Sun and nearest stars are also within it.
Like a chocolate chip in the middle of a cookie, its view is of a thin disk of cookie, other chips, and then space all around. Our Sun is one of hundreds of billions of stars embedded in a large disk.
What Elements Are Forming The Milky Way Galaxy?
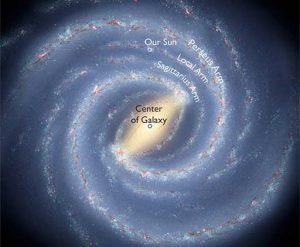
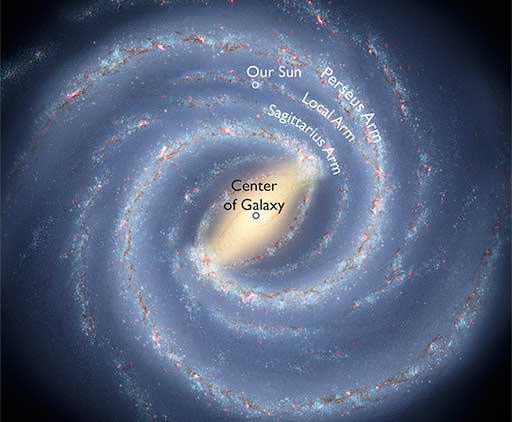
Elsewhere in deeper space, disk-shaped collections of stars are plentiful, and we refer to them as spiral galaxies. Spiral galaxies get their name for the alternating bands of bright starry and dark dusty gas swirls that pinwheel off of a bright, central core.
The arms of spiral galaxies are packed with gas and dust, and light-years of that material acts like a blanket the blocks our view beyond it. Radio waves can make their way through,
In the crowded conditions of star-forming regions in the Milky Way’s spiral arms, tightly-packed clouds of water and methanol molecules boost radio waves in the same fashion that a laser boosts light waves.
Like a sponge, these clouds soak up the incoming photons until their molecules are saturated with extra energy. Incoming photons innocently arriving into a supersoaked cloud cause an explosion of energy: As the first molecule spits out its extra energy, that energy triggers the neighboring molecule to also spit out its extra, and so on.
This cascade of energy turns the cloud into a cosmic laser that shines in microwave-length radio waves, so we call it a MASER (Microwave Amplification by Stimulated Emission of Radiation). As long as it remains close to the energy source, the cloud soaks up and releases energy constantly.
With the Very Long Baseline Array, we use masers like glowsticks to trace deeper, arm after arm, nearly through the entire thickness of the disk to construct an accurate map of our Milky Way Galaxy.
The picture we have now is a Milky Way Galaxy about 100,000 light-years across (a light-year being a distance of about six trillion miles). It has a few major arms: The Sagittarius Arm is closer to the Galactic center and the Perseus Arm is farther out in the Galaxy. Our Solar System is nestled on its own spiral arm, called the Local Arm, between these two.
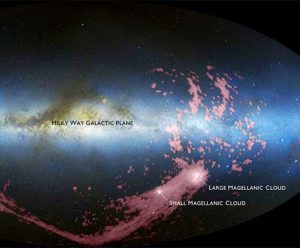
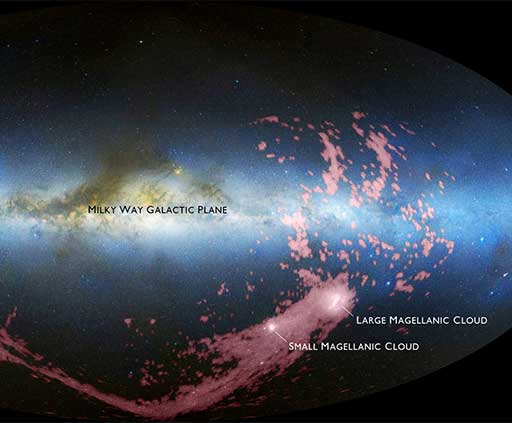
How Dust is Connecting Us to Our Milky Way Galaxy?
Dust is the atomic ash of exploded stars, the heavier molecules and elements used to make new stars, planets, and people. Inside collapsing gas and dust clouds lay countless areas of star and planet formation.
The gaseous, dusty clumps in a spiral galaxy’s arms are where the action is.
Frustratingly, dust diverts the passage of visible light, and these tantalizing regions appear like dark, impenetrable chestnuts to optical telescopes.
Radio waves, however, travel through the dusty shells of these veiled regions, so to a radio telescope, they glow clearly. Radio telescopes are the tools used to probe the hidden activities within and beyond our Milky Way Galaxy’s dusty lanes.
We see the complex, swirling feeder disks of gas and dust collecting onto a central core that will eventually start shining as a star. And because radio telescopes can measure the magnetic fields of radio objects, radio telescopes like the VLA, map the burps of excess gas traveling along the magnetic poles of young stars when they first start to shine.
Most stars in our galaxy are siblings, and the VLA has captured images of a few cloud systems where stellar siblings will soon shine.
With shorter wavelength-gathering radio telescopes, such as ALMA, we plot the particles of dust traveling around future suns. We see the traffic jams, merges, and open lanes that are evidence of planets forming in the young disk.
We can also see exactly where certain gases start to freeze out into solids, called the “snow zones.” Snow zones mark where materials can start to stick together, like snowballs, to make larger and larger bodies.
A Violent Heart At the Center of Our Milky Way Galaxy
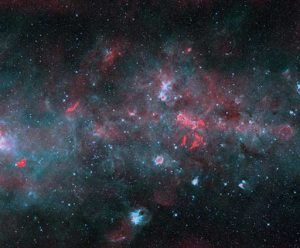
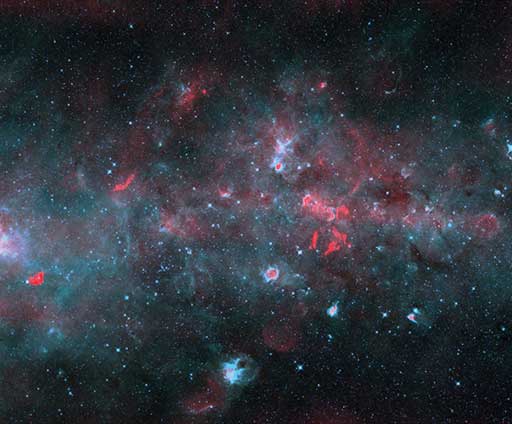
The heart of our spiral Galaxy broadcasts radio waves so powerfully that it startled an antenna engineer into discovering an entirely invisible Universe.
While identifying sources of static for wireless radio transmissions in the early 1930s, Jansky discovered a huge source of radio noise coming from what he learned to be the center of the Milky Way Galaxy, behind the constellation of Sagittarius. Later observations with more powerful radio telescopes confirmed a radio bright, compact area that has been labeled Sagittarius A.
To more finely locate the Milky Way Galaxy’s bright heart, we built a higher resolution instrument called the Green Bank Interferometer in West Virginia in the 1960s. Three antennas watched Sag A, and we combined their data to give us greater detail of the Sag A area.
We found a pinpoint of radio light within the giant Sag A area, so small that it could only be coming from a compact but powerful object, such as a black hole. The source became known as Sagittarius A*.
The VLA has since mapped the entire neighborhood around Sgr A*, seen how stars orbit at ridiculous speeds around it, spotted where its magnetic field has dragged charged gas into huge streams, and discovered where new stars manage to still form in its wake. From these observations, we now know Sgr A* to be a supermassive black hole, millions of times the mass of our Sun.
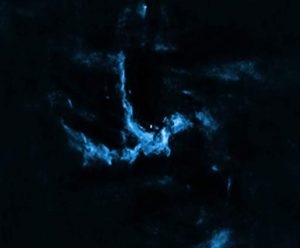
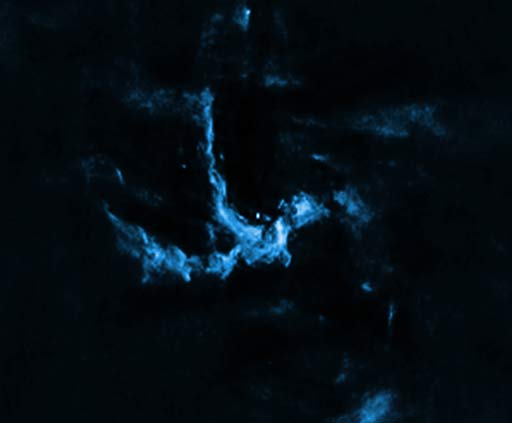
Dwarf Companions Vs Milky Way Galaxy
Like stars, galaxies seem to also form in groups. These so-called “clusters” of galaxies have a diverse range of galaxy sizes and shapes. Our Milky Way Galaxy is a member of the Local Group of galaxies whose members range from our twin spiral, Andromeda, down to little companion dwarf galaxies that hover around us.
Sky watchers in the southern hemisphere have noted these “clouds” for millennia, but only recently have we been able to determine their structure and history.
The dwarf galaxies, called the Magellanic Clouds, are roughly one-tenth the size of the Milky Way and resemble miniature versions of it. They have central cores, many bright star-forming regions, and even crude spiral structures.
Radio telescopes found that these little galaxies have a larger percentage of gas in them than the Milky Way. However, they may have had even more, as a large stream of gas points back to them from our Galaxy.
Proud makers of stars, these little galaxies likely had a burst of star-formation billions of years ago, and the collective shining of their big, new stars fountained loose gas outward. The gravity of the Milky Way snatched the stray gas and has been pulling it in ever since.