What is the VLA used for?
The Very Large Array is the most versatile, widely-used radio telescope in the world. It can map large-scale structure of gas and molecular clouds and pinpoint ejections of plasma from supermassive black holes. It is the world’s first color camera for radio astronomy, thanks to its new suite of receivers and a supercomputer than can process wide fields of spectral data simultaneously. The VLA is also a high-precision spacecraft tracker that NASA and ESA have used to keep tabs on robotic spacecrafts exploring the Solar System.
Even before its formal dedication in 1980, the VLA had become an invaluable research tool. More than 5,000 astronomers from around the world have used the VLA for more than 14,000 different observing projects. The VLA has had a major impact on nearly every branch of astronomy, and the results of its research are abundant in the pages of scientific journals and textbooks. More than 500 Ph.D. degrees have been awarded on the basis of research done with the VLA.
Discoveries
Ice on Mercury
Mercury, the innermost planet of our Solar System, is less than half the size of the Earth but is twice as close to the Sun as we are. Parts of Mercury’s Moon-like, rocky surface are heated by the Sun to temperatures nearing 800 degrees Fahrenheit (425 degrees Celsius). This is not a world like ours, certainly.
However, in 1991, planetary scientists studied Mercury using a radar system consisting of NASA’s 70-meter (230-foot) dish antenna at Goldstone, California, equipped with a half-million-watt transmitter, and the VLA as the receiving system. The VLA was configured to map Mercury with detail down to 100 meters across.
The beam of 8.5-GHz microwaves sent from Goldstone bounced off Mercury and was collected at the VLA to produce a radar image of the planet. The researchers used the Goldstone-VLA radar system to look at the side of Mercury that was not photographed by Mariner 10 in the mid-1970s.
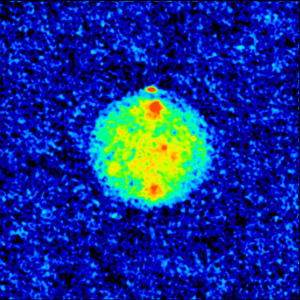

The resulting radar image, shown to the left, contained a stunning surprise. In this image, red indicates strong reflection of the radar signal and yellow, green, and blue, progressively weaker reflection. The bright red dot at the top of the image indicates strong radar reflection at Mercury’s north pole. In 1994, the team found a similar spot at Mercury’s south pole.
“Normal” ice, such as that found on Earth, is like a sponge that absorbs radio waves, but ice at very low temperatures is a mirror for radio waves. The strong reflection seen on Mercury is too large to be caused by a momentary “glint” off a crater wall, and when studied in more detail, shares the characteristics of reflections from the water ice seen on Mars and the icy moons of Jupiter.
Scientists now believe that ice collects on the floors of deep craters at Mercury’s poles, where it can remain permanently shaded from the Sun and reach temperatures as low as -235 degrees Fahrenheit (125 degrees Kelvin).
Supermassive Black Hole or Galaxy First?
In 2011, astronomers found a black hole a million times more massive than the Sun in a star-forming dwarf galaxy. The galaxy, called Henize 2-10, 30 million light-years from Earth, and is forming stars very rapidly. Irregularly shaped and about 3,000 light-years across (compared to 100,000 for our own Milky Way), it resembles what scientists think were some of the first galaxies to form in the early Universe.
Supermassive black holes lie at the cores of all “full-sized” galaxies. In the nearby Universe, there is a direct relationship — a constant ratio — between the masses of the black holes and that of the central “bulges” of the galaxies. Astronomers had concluded that black holes and their bulges affected each others’ growth.
However, in the distant Universe, looking back to a time when many more galaxies were young, astronomers found that the black holes were bigger than their host galaxies. This VLA dwarf galaxy discovery is a strong indication that supermassive black holes formed before the buildup of galaxies. This is a critical piece of the galaxy-making puzzle solved!
Microquasars
In far-distant quasars and active galaxies, millions or even billions of light-years away, the gravitational and magnetic energy of supermassive black holes is capable of accelerating “jets” of subatomic particles to speeds approaching that of light.
In the Spring of 1994, scientists were observing an X-ray emitting object called GRS 1915+105, which had just shown an outburst of radio emission. This object was known to be about 40,000 light-years away, within our own Milky Way Galaxy — in our own cosmic neighborhood. Their time series of VLA observations showed that a pair of objects ejected from GRS 1915+105 were moving apart at super speeds. This was the first time that this type of jet action had been detected in our own Galaxy.
GRS 1915+105 is thought to be a double-star system in which one of the pair is a black hole or neutron star only a few times the mass of the Sun. The more-massive object is pulling material from its stellar companion. The material circles the massive object in an accretion disk before being pulled into it. Friction in the accretion disk creates temperatures hot enough that the material emits X-rays, and magnetic processes are believed to accelerate the material in the jets we see in radio waves.
Several other Galactic “microquasars” have been discovered and studied with the VLA and the VLBA. Microquasars within our own Galaxy, because they are closer and thus easier to study than distant quasars, have become invaluable “laboratories” for revealing the physical processes that produce superfast jets of material.
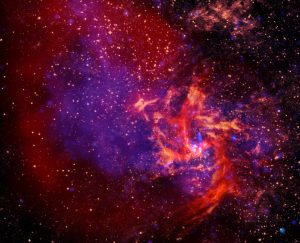
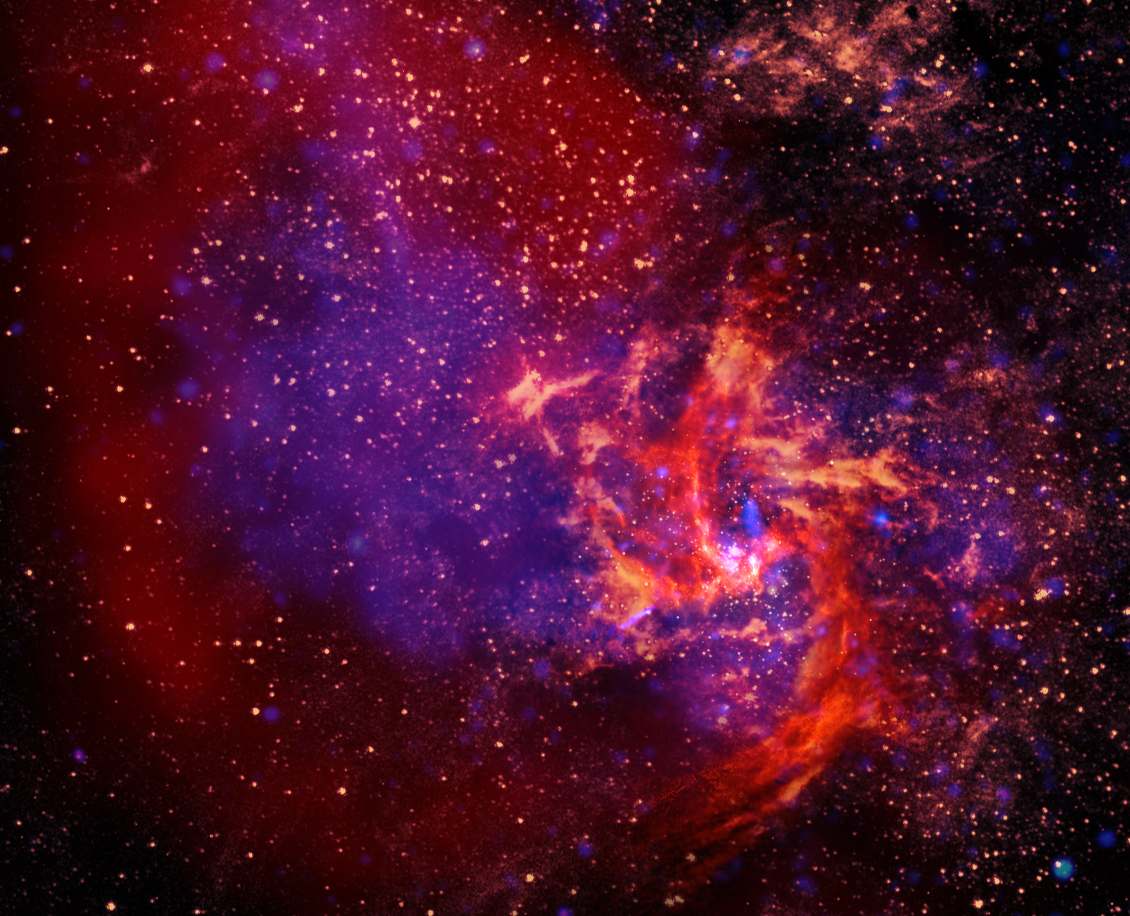
Center of our Galaxy
The complex center of our own Milky Way Galaxy is a mysterious place, shrouded by dust from the view of optical telescopes, but visible to radio telescopes such as the VLA.
In 1983, a team of observers used the VLA to make an image of the Galactic Center that revealed for the first time a “mini-spiral” of hot gas lurking there. That same year, another team made an even more-detailed image of the region that showed not only the mini-spiral but also a distinct, separate point of radio emission corresponding to the exact center of the Galaxy. That point is known as Sgr A*, discovered in 1974 by our Green Bank Interferometer in West Virginia.
From 1982 to 1998, astronomers observed Sgr A* with the VLA to measure its apparent motion around the Galaxy, if any. If Sgr A* were comparable in mass to a star, it would move rapidly around the center of the Galaxy. On the other hand, if it were a very massive object, such as a black hole, it would appear to be at rest in the Galaxy’s center.
This long-term project showed that Sgr A* appears to move very little. Observations with our more precise Very Long Baseline Array confirmed the VLA data. Today, Sgr A* is believed to be the site of a black hole about 2.6 million times more massive than the Sun.
In 1984, multiple, parallel glowing filaments were discovered at the Galactic Center, and VLA mapping projects of these complex magnetic structures are ongoing.
Einstein Rings
Albert Einstein’s General Theory of Relativity, published in 1916, predicted that massive objects, such as stars, could bend light rays passing nearby. This prediction was verified by the observation of such bending of starlight near the Sun in 1919. That same year, an English physicist, Sir Oliver Lodge, suggested that this phenomenon could produce a gravitational lens.
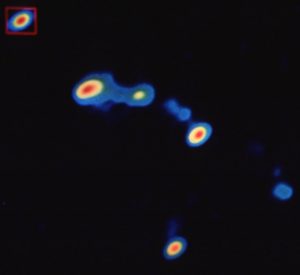
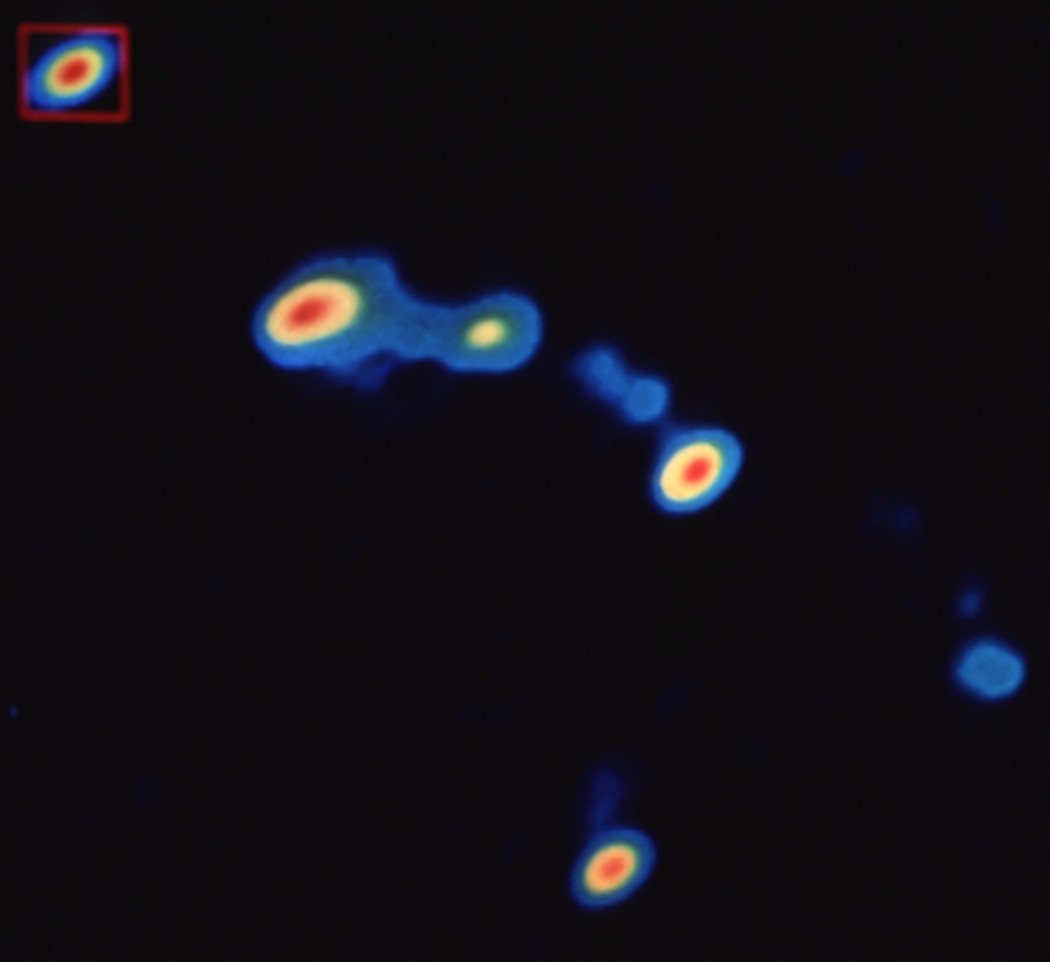
In 1936, Einstein himself showed that, if a brightly-emitting object were exactly behind a massive body capable of making a gravitational lens, the result would be an image of a ring around the massive lensing object. However, he dismissed the possibility of ever discovering such a lens, because of the small chance that the precise coincidence required would ever arise.
Optical observers discovered the first gravitational lens in 1979, and the VLA quickly was used to confirm the discovery (shown to the left). The fourth known gravitational lens was discovered with the VLA.
In early 1987, a group of observers made short, two-minute, “snapshot” images of a large number of radio-emitting objects with the VLA. An object known as MG1131+0456 showed an interesting oval structure, with elongated bright spots at either end. Thus, more than 50 years after Einstein’s prediction, the VLA discovered the type of object he had considered unlikely ever to be found. Subsequent studies have shown that this object consists of a distant quasar whose radio emission is being bent, or “lensed” by a galaxy between the quasar and Earth.
Nature of Gamma-ray Bursts
Gamma Ray Bursts (GRBs) were discovered in 1967 by satellites designed to monitor compliance with the atmospheric nuclear test ban treaty. The short-lived outbursts of the most energetic electromagnetic radiation remained one of the biggest mysteries in astrophysics for nearly three decades thereafter. For most of that period, the bursts’ positions in the sky were known only with limited precision, making study of them by ground-based optical and radio telescopes impossible.
Because of the distance uncertainty, for example, astronomers did not know if GRBs were in our own Solar System, our Galaxy, or in the distant Universe.
On May 8, 1997, the Italian-Dutch satellite BeppoSAX detected a Gamma Ray Burst, and VLA observers discovered radio emission coming from this object on May 13. Since then, the VLA has been used to follow several GRB “afterglows.”
While GRB afterglows fade quickly at other wavelengths, the VLA has been able to follow an afterglow for more than a year, tracking changes in its intensity and other characteristics. These observations reveal the size of the fireball and the speed of its expansion.
After three decades of mystery, astronomers now know that GRBs, the most violent events in the current Universe, occur in the dusty parts of galaxies, where it is likely that young and/or massive stars still reside within the clouds from which they formed. This evidence supports the theory that GRBs result from a “hypernova,” the explosive death of a very massive star that collapses and forms a black hole.